Membrane Steam Methane Reforming: A Breakthrough for Low-Carbon Hydrogen
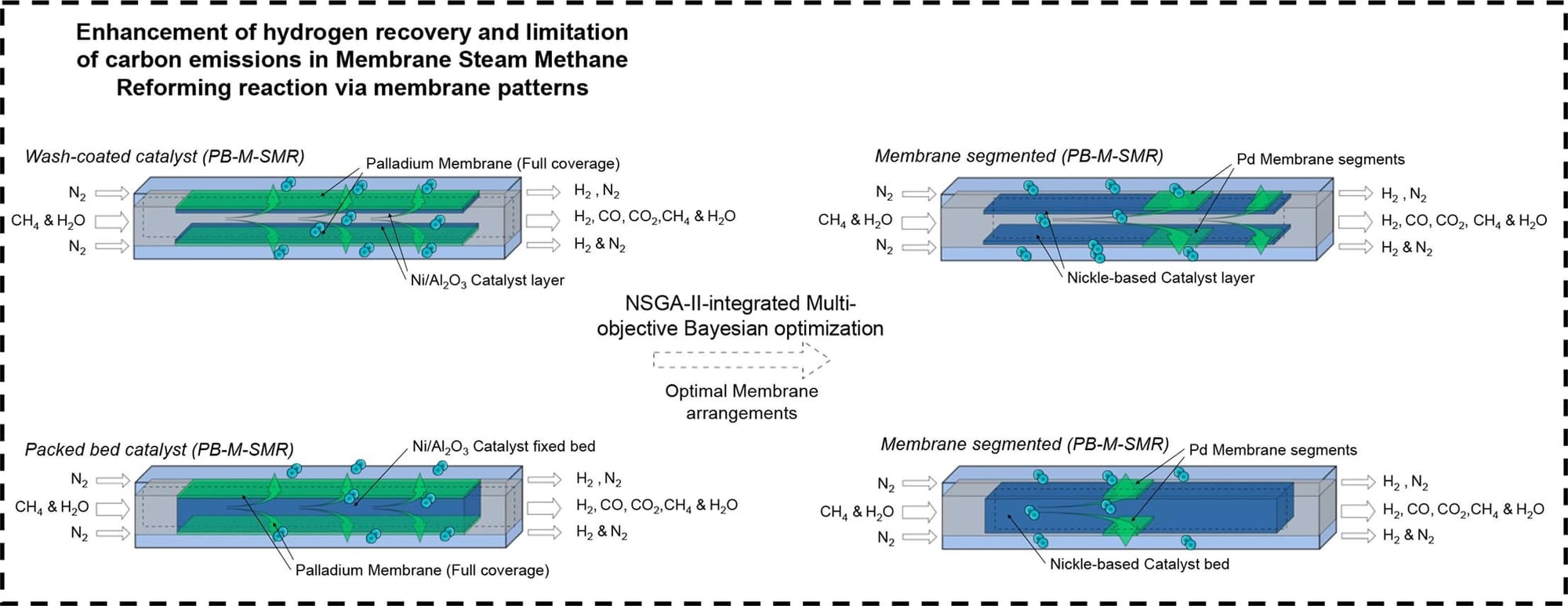
An article published by Ali Cherif et al on Energy Conversion and Management titled, "Low-carbon membrane steam methane reforming: Unveiling the trade-off between environmental impact and performance through multi-objective Bayesian optimization" explores a novel method to mitigate carbon emissions during hydrogen production. This research delves into the viability of membrane steam methane reforming (M-SMR), a process integrating a Pd-based membrane for hydrogen separation within the conventional steam methane reforming (SMR) reactor. The study specifically focuses on comparing two reactor designs: a wash-coated catalytic reactor and a packed-bed reactor. Additionally, the authors employed a multi-objective Bayesian optimization (MOBO) approach to optimize the performance, environmental impact, and cost of this new M-SMR process.
The researchers establish the potential of M-SMR for clean hydrogen production, arguing its ability to drastically reduce CO2 emissions compared to traditional SMR. They highlight the role of the membrane, which selectively allows hydrogen to pass through, facilitating its separation from the byproduct CO2. This captured CO2 offers avenues for sequestration or utilization, aligning with the goals of carbon-conscious fuel production. Moreover, the article underscores the significance of reactor configuration, with experiments demonstrating that the packed-bed reactor outperforms the wash-coated design. This superior performance is attributed to factors like improved mass transfer rates and higher catalyst loading. The authors' utilization of the MOBO technique is particularly noteworthy as it allowed them to establish optimal operating conditions and membrane properties, balancing the trade-offs between performance indicators and environmental sustainability.
The article meticulously outlines the methodology employed by the research team. Theoretical modeling played a vital role in simulating the integrated M-SMR system with its complex interplay of chemical reactions, heat transfer, and mass transport phenomena. The MOBO approach, empowered by machine learning algorithms, facilitated intelligent exploration of a vast parameter space, ultimately identifying the best configurations for maximizing hydrogen output, minimizing carbon emissions, and ensuring cost-competitiveness. Despite the promising findings, the study also acknowledges the limitations of M-SMR. Increased pressure drops within packed-bed reactors pose a challenge in process design, and the long-term stability of membranes under industrial conditions necessitates further scrutiny. Nonetheless, this research represents a significant advancement in the pursuit of sustainable hydrogen production technologies, highlighting the potential of M-SMR systems to facilitate the transition towards a clean energy future.